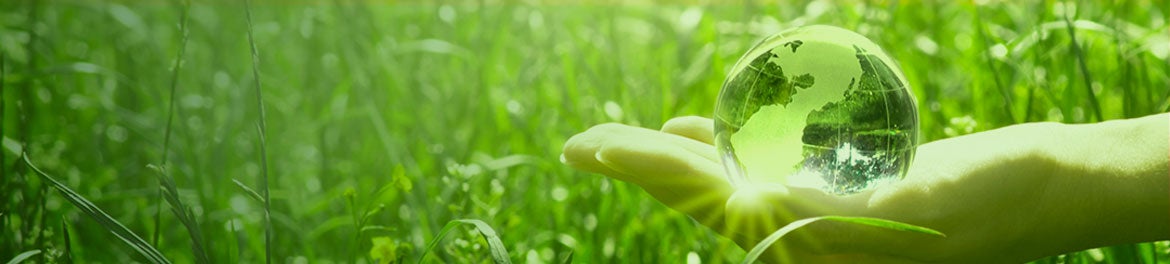
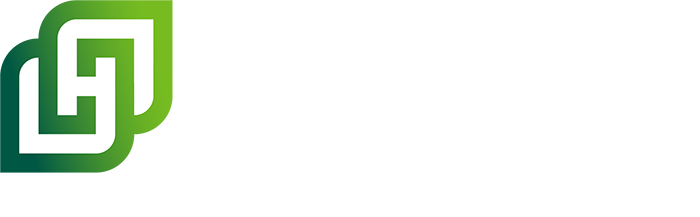
Foreword and Executive Summary - Electrifying Heat in an Existing Hospital
Providence St. Peter Hospital Decarbonization Case Study
Foreword
Recognizing the growing interest in decarbonizing the health care sector, the American Society for Health Care Engineering (ASHE) sought to support its members wishing to decarbonize their facilities, and in April 2023 ASHE began discussions with Providence about the need for a comprehensive case study to examine the feasibility of decarbonizing an existing hospital building. There had been extensive effort on guidance for decarbonizing new hospital buildings, but little information was available about the decarbonization of an existing hospital building. Additionally, there was speculation about the feasibility of decarbonizing an existing hospital from a technical and financial perspective.
As ASHE explored the concept of sponsoring a case study and investigating the feasibility of decarbonizing an existing hospital building, Providence was identified as a key partner due to its leadership in environmental sustainability and its desire to share lessons learned broadly with the field. Providence St. Peter Hospital (PSPH), a community hospital in Olympia, Wash., was selected for the case study project. The hospital is 733,000 square feet and has 372 licensed beds, and its first phase was built in 1969 during the Hill-Burton era when thousands of health care facilities were built nationwide. Geographical location and climate were considered when selecting a case study hospital and the mild climate of Olympia, Wash., was considered appropriate since it represents a baseline case study demonstrating the feasibility of decarbonizing in a temperate geographical location. In addition, the fact that PSPH was ahead of many others on its journey toward decarbonization was considered. The hospital has robust utility and building metering and utilizes 100% renewable power. As a health care system, Providence has developed a climate action plan that incorporates decarbonization strategies for anesthetic gases, transportation, waste and electricity. For PSPH, the missing piece from the overall decarbonization strategy was the thermal energy load. For that reason, the ASHE study investigated decarbonizing the thermal load specifically.
The research for the case study took place over a year, and there were several key takeaways identified. First, it is technically feasible for PSPH to decarbonize the thermal load. However, due to the nature of the hospital as a patient care environment and the need for normal operations, it will take 10 to 15 years to achieve carbon neutrality for the thermal load. This is an important factor because it demonstrates the need for careful planning and early preparation as organizations seek to decarbonize.
Second, for this hospital it is financially feasible to decarbonize the thermal load. The study estimated that full electrification of the thermal load would cost around $100/square foot in capital expenditures. Several financial mechanisms are available today that might help PSPH move forward with electrification, such as the incentives in the Inflation Reduction Act of 2022.
Finally, it is noteworthy that technologies for decarbonization are emerging, continually changing and moving very quickly. Equipment that is on the cutting edge today will be out of circulation by the time the thermal system is fully electrified. This is a critical consideration and emphasizes the need to plan early and be mindful that technologies will shift.
The case study provides a detailed outline of the steps one hospital is taking to achieve this feat. Yet, to be clear, this publication is not intended to be a playbook for electrifying any given hospital, nor is it meant to be a benchmark by which other hospitals pursuing this goal should be measured. The path to complete electrification is an extremely complex and bespoke process. Each electrification plan must be tailored to the specific goals of a given health care facility or system as well as regulatory requirements that govern that facility and the needs of the communities it serves.
As a mechanical engineer who has built my career around environmental stewardship and sustainability in health care, this case study project, while a huge endeavor, was also a labor of love and a pleasure to be a part of. I would like to thank Fred Betz, Ph.D., for his tireless effort in leading the study as well as other contributors including Peter Dahl, define sustainability; Sagar Rao, NeuMod Labs; Walt Vernon, Mazzetti; and Kyle Victor, McKinstry. Other key contributors include Yousif Alshaba, Scott Acker and Mark Thynes, McKinstry; Jennifer Ashlock, Puget Sound Energy; Jeff Probst, Konvekta; and Tom Gelin, Air Flow, Inc. A huge thank you goes to the Providence Southwest Washington Foundation’s Environmental Stewardship Fund and the Washington State Society for Healthcare Engineering, whose gracious donations to support this important study ensured its completion. I would also like to thank my friends at Providence, including Ali Santore, Elizabeth Schenk, Ph.D., Dave Thomsen and Geoffrey Glass, for their leadership and commitment to the project. Lastly, I want to express my gratitude to the PSPH facilities team, including Clay Ciolek and Gregory Pries, whose dedication and expertise proved exceedingly valuable.
It is my hope that this case study will serve as a success story, meant to inspire and demonstrate that this feat, which not so long ago seemed impossible, might be, in fact, within reach. If hospital electrification were a competitive sport, PSPH would be an Olympic gold medalist. No doubt, the hospital’s incredible efforts have set a new standard and raised the caliber of what is possible in sustainability. While not every hospital can be held to the standard set by PSPH, their example should encourage more health care facilities to try. As they say, a rising tide lifts all boats.
Kara Brooks, MS, LEED AP BD+C, Senior associate director of sustainability, AHA
Executive Summary
The American Society for Health Care Engineering (ASHE) funded a decarbonization feasibility case study at Providence St. Peter Hospital (PSPH) in Olympia, Wash., with the goal of verifying the technical and financial feasibility of achieving Scope 1 and Scope 2 carbon neutrality at PSPH.1 This facility was selected as a typical large inpatient hospital that represents a reasonable reference for the health care industry. The operations and unique features of hospitals, such as requiring steam for sterilization, makes them more complex to electrify than many other commercial buildings.
Two major challenges are addressed in this study: (1) determining pathways to achieve electrification of the heating plant while maintaining a resilient supply of energy and supporting a full service of operations and (2) determining the impact on the electrical utility supply to achieve beneficial electrification. The goal for PSPH is to eliminate combustion-based Scope 1 emissions by electrifying the heating plant that currently exists: two dual-fueled boilers. This goal also includes discontinuing the natural gas supply to eliminate the associated upstream methane leaks and complements the 100% renewable electricity already being purchased by the hospital.
The approach to assessing and decarbonizing PSPH was to carefully analyze the existing conditions, the energy use and peak heating demand by end-use. The next step was to identify approaches to reduce heating loads through investments in certain technologies and account for the overall impact. A calibrated energy model was developed per ASHRAE Guideline 14, Measurement of Energy, Demand, and Water Savings, requirements to fill in any data gaps and to further support energy and financial analyses.2 The recommended strategy is based on a systems-thinking approach to address as many demand-side energy savings measures as feasible to reduce the plant load. The installed heating capacity is 25 million British thermal units (Btu) per hour (MMBtu/hr) or 7,327 kilowatts (kW), and the measured peak load was 20 MMBtu/hr (5,861 kW), which can be reduced to 11 MMBtu/hr (3,224 kW) if all demand-side measures are implemented. The reduced heating load will enable greater flexibility on the heating hot water (HHW) distribution side in terms of smaller pipe sizes and lower temperatures. The target peak HHW temperature is 140 F to achieve efficient heat pump operation. However, this is dependent on the level of implementation of demand-side measures, especially on the envelope upgrades. Upon completion of the distribution system upgrades, the heating plant can be upgraded with air-source heat pumps as the primary source of thermal energy for normal operation and augmented with a heat recovery chiller and 100,000 gallons of HHW storage.
The implementation of the decarbonization strategy will need to be phased and may take several years to implement depending on available resources.
The recommended phasing is as follows:
- Demand-side upgrades (nine-year duration).
- Implement air-side heat recovery upgrades.
- Add insulation and glazing upgrades.
- Upgrade process steam-using systems (kitchen and sterile processing areas).
- Continue implementing other energy savings measures.
- Distribution system upgrades (one- to two-year duration).
- Migrate loads to existing HHW pipes after demand-side upgrades are completed.
- Replace steam pipes with HHW pipes (starting in springtime to have seven months of low heating demand before cold weather arrives in winter).
- Replace steam converters with heat exchangers for service hot water loops.
- Add variable primary HHW pumping system.
- Plant system upgrades (three- to five-year duration).
- Add a south expansion or penthouse to the central utility plant to house the air source heat pumps.
- Upgrade electrical infrastructure, add generators and add generator jacket heat recovery.
- Install air-source heat pumps.
- Decommission boilers.
- Install thermal storage or microgrid in boiler footprints.
There are two somewhat unique features at PSPH that simplify the electrification and decarbonization process. First, the hospital does not have humidification due to the marine climate not requiring it. Relative humidity data was analyzed that demonstrated compliance with a minimum 30% relative humidity for all hours with excursions below 30% for less than 12 hours per year. The relative humidity did not fall below 20%. Second, the service hot water (SHW) system operates at 120 F, which simplifies serving the SHW from the HHW loop rather than either running a warmer HHW loop or applying a stand alone heat pump system. Although it is more common to operate SHW systems at 140 F per Centers for Disease Control and Prevention guidance, no legionella concerns have arisen at PSPH at the lower temperatures.
The resilience strategy is to maintain on-site diesel fuel storage that can support generators and boilers in the near term. The generators will be upgraded to include engine jacket water heat recovery to support the heating demand during a power outage when the plant is upgraded. The heat recovery effectively turns the generators into a combined heat and power system providing both electrical and thermal energy to the hospital during outages. The boilers will stay in place until operational proficiency is achieved with the heat pump plant and generator heat recovery. Current testing and emergency power use accounts for approximately 4% of annual on-site combustion emissions. Resilience solutions such as thermal storage and microgrids will be reevaluated to replace the boilers as the technology matures.
Microgrids are being implemented in a few hospitals today that can be referenced for this study and should align with this project’s timeline. For now, on-site fuel storage is being accepted as a resilience solution as it is a small emission source due to few run hours and it assists in eliminating the natural gas connection that results in methane leaks.
The study investigated four potential scenarios for the hospital between 2024 and 2041 based on a regulatory framework of the recently passed Seattle Building Emissions Performance Standard.
- Scenario 0 is a business-as-usual case where the hospital accepts a onetime $7.5 million fine and makes incremental capital improvements over time.
- Scenario 1 is the minimum disruption scenario that only replaces the existing dual-fuel boilers with two electric resistance boilers plus supporting generators and leaves the balance of the systems in place.
- Scenario 2 implements demand-side energy savings measures on 100% outside air systems, electrified process loads with heat pumps or standalone electric boilers and a host of other demand-side energy savings measures. An air-source heat pump plant would then be implemented to support the 16 MMBtu/hr (4,689 kW) heating load.
- Scenario 3A adds envelope energy savings measures to replace single-pane windows and insulation to uninsulated or partially insulated walls. The envelope measures reduce the heating load to 11 MMBtu/hr (3,224 kW).
- Scenario 3B was broken out to isolate the impact of the insulation improvements as it is a costly upgrade with a poor return on investment.
A third-party cost estimate was developed to inform the three decarbonization scenarios. A detailed list of systems, components and materials is described in Chapter 7, Capital Equipment and Costs. The capital cost for Scenario 1 was estimated to be $57,711,000, or $79/ft2, for Scenario 2 was estimated to be $62,171,000, or $85/ft2, for Scenario 3A was estimated to be $80,425,000, or $110/ft2, and for Scenario 3B was estimated to be $68,400,000, or $93/ft2. For reference, a full tenant improvement (TI) on a hospital is between $100/ft2 and $150/ft2. However, the square footage is the entirety of the hospital or 733,000 ft2. Also, the TI is nearly exclusively focused on infrastructure enhancements, which is atypical for a TI.
A cost model using 2024 dollars was built for a 17-year time frame that compiled capital cost, energy cost and carbon fines using the cost estimate, energy models and an estimate of a future Olympia, Wash., carbon fine based on the current Seattle Building Emissions Performance Standard fine structure. The cost results are compiled in the following figure.
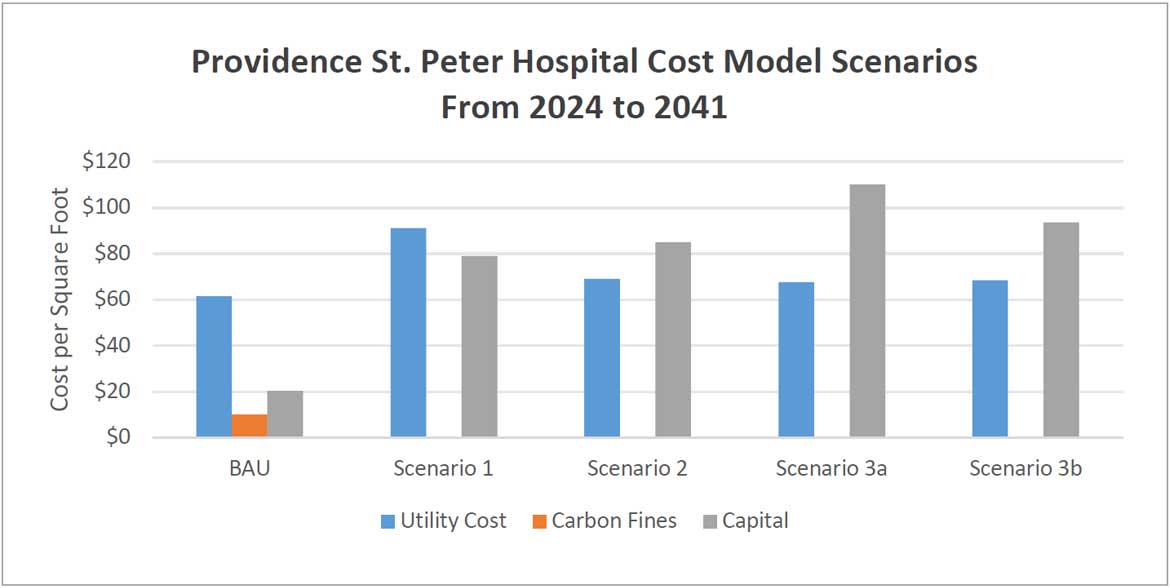
The total cost of ownership over 17 years for each case is as follows:
- Scenario 0: $67.2 million ($92/ft2)
- Scenario 1: $124.2 million ($169/ft2)
- Scenario 2: $112.6 million ($154/ft2)
- Scenario 3A: $130.0 million ($177/ft2)
- Scenario 3B: $118.4 million ($162/ft2)
The most cost effective fully electrified solution is Scenario 2, though it is still $45 million more costly over 17 years than Scenario 0, the business-as-usual case. It is worth noting that this study does not include utility rate escalations or utility incentives. Furthermore, some of the capital equipment, such as heat pumps, may decline in cost as the market matures. More cost details are available in Section 2.4.
Notes
- EPA. “Scope 1 and Scope 2 Inventory Guidance.” EPA Center for Corporate Climate Leadership.
- ASHRAE. ASHRAE Technology Portal.